The Mouse Trap
Text-only version.
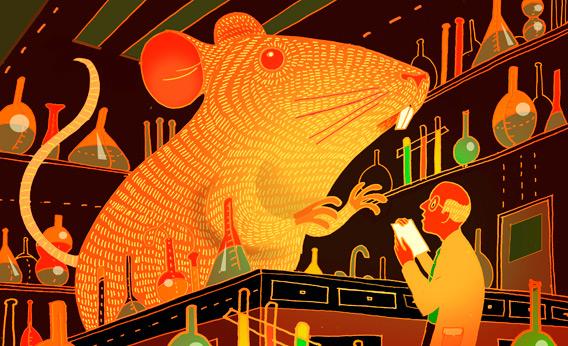
Illustration by Rob Donnelly.
See this series in its original version, with all of the accompanying images and videos: Part I, Part II, Part III.
Part I: The Mouse Trap: The dangers of using one lab animal to study every disease.
Mark Mattson knows a lot about mice and rats. He's fed them; he's bred them; he's cut their heads open with a scalpel. Over a brilliant 25-year career in neuroscience—one that's made him a Laboratory Chief at the National Institute on Aging, a professor of neuroscience at Johns Hopkins, a consultant to Alzheimer's nonprofits, and a leading scholar of degenerative brain conditions—Mattson has completed more than 500 original, peer-reviewed studies, using something on the order of 20,000 laboratory rodents. He's investigated the progression and prevention of age-related diseases in rats and mice of every kind: black ones and brown ones; agoutis and albinos; juveniles and adults; males and females. Still, he never quite noticed how fat they were—how bloated and sedentary and sickly—until a Tuesday afternoon in February 2007. That's the day it occurred to him, while giving a lecture at Emory University in Atlanta, that his animals were nothing less (and nothing more) than lazy little butterballs. His animals and everyone else's, too.
Mattson was lecturing on a research program that he'd been conducting since 1995, on whether a strict diet can help ward off brain damage and disease. He'd generated some dramatic data to back up the theory: If you put a rat on a limited feeding schedule—depriving it of food every other day—and then blocked off one of its cerebral arteries to induce a stroke, its brain damage would be greatly reduced. The same held for mice that had been engineered to develop something like Parkinson's disease: Take away their food, and their brains stayed healthier.
How would these findings apply to humans, asked someone in the audience. Should people skip meals, too? At 5-foot-7 and 125 pounds, Mattson looks like a meal-skipper, and he is one. Instead of having breakfast or lunch, he takes all his food over a period of a few hours each evening—a bowl of steamed cabbage, a bit of salmon, maybe some yogurt. It's not unlike the regime that appears to protect his lab animals from cancer, stroke, and neurodegenerative disease. "Why do we eat three meals a day?" he asks me over the phone, not waiting for an answer. "From my research, it's more like a social thing than something with a basis in our biology."
But Mattson wasn't so quick to prescribe his stern feeding schedule to the crowd in Atlanta. He had faith in his research on diet and the brain but was beginning to realize that it suffered from a major complication. It might well be the case that a mouse can be starved into good health—that a deprived and skinny brain is more robust than one that's well-fed. But there was another way to look at the data. Maybe it's not that limiting a mouse's food intake makes it healthy, he thought; it could be that not limiting a mouse's food makes it sick. Mattson's control animals—the rodents that were supposed to yield a normal response to stroke and Parkinson's—might have been overweight, and that would mean his baseline data were skewed.
"I began to realize that the ‘control’ animals used for research studies throughout the world are couch potatoes," he tells me. It's been shown that mice living under standard laboratory conditions eat more and grow bigger than their country cousins. At the National Institute on Aging, as at every major research center, the animals are grouped in plastic cages the size of large shoeboxes, topped with a wire lid and a food hopper that's never empty of pellets. This form of husbandry, known as ad libitum feeding, is cheap and convenient since animal technicians need only check the hoppers from time to time to make sure they haven’t run dry. Without toys or exercise wheels to distract them, the mice are left with nothing to do but eat and sleep—and then eat some more.
That such a lifestyle would make rodents unhealthy, and thus of limited use for research, may seem obvious, but the problem appears to be so flagrant and widespread that few scientists bother to consider it. Ad libitum feeding and lack of exercise are industry-standard for the massive rodent-breeding factories that ship out millions of lab mice and rats every year and fuel a $1.1-billion global business in living reagents for medical research. When Mattson made that point in Atlanta, and suggested that the control animals used in labs were sedentary and overweight as a rule, several in the audience gasped. His implication was clear: The basic tool of biomedicine—and its workhorse in the production of new drugs and other treatments—had been transformed into a shoddy, industrial product. Researchers in the United States and abroad were drawing the bulk of their conclusions about the nature of human disease—and about Nature itself—from an organism that's as divorced from its natural state as feedlot cattle or oven-stuffer chickens.
Mattson isn't much of a doomsayer in conversation. "I realized that this information should be communicated more widely," he says without inflection, of that tumultuous afternoon in Atlanta. In 2010, he co-authored a more extensive, but still measured, analysis of the problem for the Proceedings of the National Academy of Sciences. The paper, titled " 'Control' laboratory rodents are metabolically morbid: Why it matters," laid out the case for how a rodent obesity epidemic might be affecting human health.
Standard lab rats and lab mice are insulin-resistant, hypertensive, and short-lived, he and his co-authors explained. Having unlimited access to food makes the animals prone to cancer, type-2 diabetes, and renal failure; it alters their gene expression in substantial ways; and it leads to cognitive decline. And there's reason to believe that ragged and rundown rodents will respond differently—abnormally, even—to experimental drugs.
Mattson has seen this problem in his own field of research. Twenty years ago, scientists started to develop some new ways to prevent brain damage after a stroke. A neurotransmitter called glutamate had been identified as a toxin for affected nerve cells, and a number of drug companies started working on ways to block its effects. The new medicines were tested in rats and mice with great success—but what worked in rodents failed in people. After a series of time-consuming and expensive clinical trials, the glutamate-blockers were declared a bust: They offered no benefit to human stroke patients.
Now Mattson has an idea for why the drugs didn't pan out: All the original test-animals were chubby. If there's something about the brain of an obese, sedentary rodent that amplifies the effects of a glutamate-blocker, that would explain why the drugs worked for a population of lab animals but not in the more diverse set of human patients. This past June, he published a paper confirming the hunch: When he put his test mice on a diet before administering the glutamate-blockers, the drugs' magical effects all but disappeared.
Many promising treatments could be failing for the same reason, Mattson argues, and other trials should be re-examined—but that's unlikely to happen anytime soon. "It comes down to money and resources," he says. "There's some fraction of studies that may have been compromised by [these] issues, but there's no way to know unless one does the experiment with the proper controls."
That's the drawback of the modern lab mouse. It's cheap, efficient, and highly standardized—all of which qualities have made it the favorite tool of large-scale biomedical research. But as Mattson points out, there's a danger to taking so much of our knowledge straight from the animal assembly line. The inbred, factory-farmed rodents in use today—raised by the millions in germ-free barrier rooms, overfed and understimulated and in some cases pumped through with antibiotics—may be placing unseen constraints on what we know and learn.
"This is important for scientists," says Mattson, "but they don't think about it at all."
* * *
Mattson is not the only one with doubts, nibbling away at the corner of his cage. The rise of the factory mouse has implications that extend far beyond his work on Parkinson's disease and stroke. By focusing so intently on one organism, raised in a certain way, we may be limiting our knowledge of cancer, too, and heart disease, and tuberculosis—the causes of death for many millions of people every year. If Mattson is right, science may be faced with a problem that is mind-boggling in its scope. Funding agencies in the United States and Europe will spend hundreds of millions of dollars in the coming years to further fiddle with and refine the standard organism, doubling down on a bet that goes back at least six decades: Establishing a single animal as the central determinant of how we study human illness, design new medicines, and learn about ourselves.
Just how ubiquitous is the experimental rodent? In the hierarchy of lab animal species, the rat and mouse rule as queen and king. A recent report from the European Union counted up the vertebrates used for experiments in 2008—that's every fish, bird, reptile, amphibian, and mammal that perished in a research setting, pretty much any animal more elaborate than a worm or fly—and found that fish and birds made up 15 percent; guinea pigs, rabbits, and hamsters contributed 5 percent; and horses, monkeys, pigs, and dogs added less than 1 percent. Taken together, lab rats and lab mice accounted for nearly all the rest—four-fifths of the 12 million animals used in total. If you extend those proportions around the world, the use of rodents is astonishing: Scientists are going through some 88 million rats and mice for their experiments and testing every year.
Dead mice pile up more than three times faster than dead rats, which makes sense given their relative size. Cheap, small animals tend to be killed in greater volumes than big ones. A researcher might run through several dozen mice, half a dozen rabbits, or a pair of monkeys to achieve the same result: one published paper. More striking, then, is the extent to which papers about rats and mice—however many animals go into each—dominate the academic literature. According to a recent survey of animal-use trends in neuroscience, almost half the journal pages published between 2000 and 2004 described experiments conducted on rats and mice.
A survey of the National Library of Medicine's database of more than 20 million academic citations shows the same trend across the whole of biomedicine. Since 1965, the number of published papers about dogs or cats has remained fairly constant. The same holds true for studies of guinea pigs and rabbits. But over that 44-year stretch, the number of papers involving mice and rats has more than quadrupled. What about the simpler organisms that researchers tend to poke and prod—yeast and zebra fish and fruit flies and earthworms? By 2009, the mouse itself was responsible for three times as many papers as all of those combined.
That is to say, we've arrived at something like a monoculture in biomedicine. The great majority of how we understand disease, and attempt to cure it, derives from a couple of rodents, selected—for reasons that can seem somewhat arbitrary in retrospect—from all the thousands of other mammals, tens of thousands of other vertebrates, and millions of other animal species known to walk or swim or slither the Earth. We've taken the mouse and the rat out of their more natural habitats, from fields and barns and sewers, and refashioned them into the ultimate proxy for ourselves—a creature tailored to, and tailored by, the university basement and the corporate research park.
It's just the latest step in a trend that began more than a century ago. The splendid menagerie that once formed the basis for physiological study—the sheep, the raccoons, the pigeons, the frogs, the birds, the horses—has since the early 1900s been whittled down to a handful of key "model systems": Animals that are special for not being special, that happen to flourish under human care and whose genes we can manipulate most easily; the select and selected group that are supposed to stand in for all creation. Where scientists once tried to assemble knowledge from the splinters of nature, now they erect it from a few standardized parts: An assortment of mammals, some nematodes and fruit flies, E. coli bacteria and Saccharomyces yeast. Even this tiny toolkit of living things has in recent decades been shrunk down to a favored pair, the rat and mouse. The latter in particular has become a biological Swiss army knife—a handyman organism that can fix up data on cancer, diabetes, depression, post-traumatic stress, or any other disease, disorder, or inconvenience that could ever afflict a human being. The modern lab mouse is one of the most glorious products of industrial biomedicine. Yet this powerful tool might have reached the limit of its utility. What if it's taught us all it can?
* * *
The government's top researcher on tuberculosis—still one of the world's most deadly infections—seems to be running a midsized wildlife park out of his Maryland home. In a modest house on a tree-lined street in Germantown, Clif Barry keeps two kinds of turtles, three veiled chameleons, two Jackson's chameleons, six species of frogs, half a dozen fish tanks (filled with cichlids, goldfish, and piranhas, kept separately), two dogs (named Jacques and Gillian), and an Australian tree python. "I'm an animal person," he tells me. "My house would require a zookeeper's license if Montgomery County knew what I had."
Twenty miles away in Bethesda, though, where Barry serves as chief of the Tuberculosis Research Section at the National Institute of Allergy and Infectious Diseases, a single animal has taken over the ecosystem. It has infested every paper and conference, and formed a living, writhing barrier to new drugs on their way to clinical trials. "We've always only tested things in mice," Barry tells me by phone one afternoon. "The truth is that for some questions, mice give you a very nice and easy model system for understanding what's happening in humans, but mice are mice, and people are people. If we look to the mouse to model every aspect of the disease for man, and to model cures, we're just wasting our time."
The problem, he says, begins with the three M’s. The process of drug discovery has been carried out in the same way for decades. You start by testing a new compound in a Petri dish, to find out whether it can slow the growth of a particular bacterium in culture. That gives you the smallest dose that has an effect, known as the minimum inhibitory concentration, or "MIC"—the first M. Then you move to a living animal: Does the compound have any effect on the course of disease in a lab mouse? If so, you've cleared the second M, and you're ready to test the compound in the third M, man. Each step leads to the next: No drug can be tested in man until it's been shown to work in mice, and no drug is tested in mice until it's been shown to have a reasonable effect in the dish. "The bad part of that," says Barry, "is that no part of it is predictive:" A new compound that succeeds in the dish might flunk out in the mouse, and something that can cure tuberculosis in a mouse could wash out in people.
Take the example of pyrazinamide, one of the front-line drugs in the treatment of tuberculosis. Along with three other antibiotics, it forms the cocktail that remains, despite ongoing research, our only way of defeating the infection. But pyrazinamide didn't make it through the three Ms: It does nothing in the dish—there's no MIC whatsoever—and it has a weak effect in mice. According to Barry, if a compound like that were discovered in 2011, it would never make its way into clinical trials. Forty years ago, the system wasn't so rigid. A prominent physician and researcher at Britain's Medical Research Council named Wallace Fox saw something intriguing in the animal data: Pyrazinamide's action seemed to persist when those of other drugs had stopped. He insisted on testing the drug in humans, and its effects were profound. The fact that nothing gets to humans today without first passing the mouse test, says Barry, "has cost us a new generation of medicines."
Indeed, there's been no real breakthrough in treating tuberculosis—no major pharmaceutical discoveries—since the early 1970s. The first antibiotic to have any success against the tuberculosis mycobacterium, the first that could penetrate its waxy coating, was discovered (and tested in guinea pigs) in the early 1940s. The best vaccine we have was first used in humans in 1921. (It works pretty well against severe childhood forms of the disease, but less so otherwise.) And the closest thing we have to a miracle cure—the multidrug cocktail that doesn’t work against every strain and requires a six-month course of treatment with severe side effects—was finalized during the Nixon administration. Since then, almost every new idea for how to treat TB has come from experiments on lab mice. These have given us enough new data to drown the infected in a tsunami of graphs and tables, to bury them in animal carcasses. Yet we've made little progress—OK, no progress at all—in treating the human disease. Tuberculosis causes more than 2 million deaths every year, and we're using the same medicines we had in 1972.
One major problem with the mouse model—and the source of its spotty track record in the clinic—is well-known among those in the field: The form of TB that mice happen to get isn't all that much like our own. A human case of the disease begins when infectious bacilli are inhaled into the lungs, where they grow in number as the immune system sends in its soldiers to fight them off. White blood cells swarm the bacteria in a rolling, alveolar scrum, forming a set of pearly-white masses the size of golf balls called granulomas. These are where the war between body and invader plays out in a series of contained skirmishes.
As more immune cells are recruited to fight off the infection, some of the balls swell and stratify into a more developed form: A sphere of macrophages and lymphocytes packed inside a fibrous shell, with a cottage cheese clump of dead cells and bacteria at its core. At this point the battle reaches a stalemate: The bacteria stop dividing; the body has controlled, but not eliminated, the infection. For most people who have the disease, it's a ceasefire that holds indefinitely.
But for some patients a latent case of tuberculosis can suddenly become active. The granulomas rupture and propagate, spilling thousands of organisms into the lungs, where they can be aerosolized, coughed up, and passed on to a new host. Left untreated, the infection migrates into the bloodstream and other organs; widespread inflammation leads to burst arteries or a ruptured esophagus; and in about half of all cases, the patient dies.
The layered granuloma is the defining feature of human tuberculosis: The place where the host fights the infection (successfully or not), and the necessary site of action for any drug. To cure the disease, a treatment must be able to penetrate each ball of cells, whatever its type or composition; every last bacterium must be destroyed. "It's the structure of those granulomas that makes it so difficult to treat TB," says Barry. And they simply don't exist in mice.
If you infect a mouse with TB—if you spritz a puff of infected air into its nostrils through a trumpet, as so many labs do around the world—the animal's lungs quickly fill up with bacteria and immune cells, like a nasty case of pneumonia. There are no discrete balls of tissue, no well-defined granulomas sheathed in fibrin, no array of structures that harbor the bugs at various stages of development. The mice have no special, latent form of TB, either, and no way to pass on the disease. They simply die, after a year or two, of a slow and progressive decline.
That's why we've made so little progress using mice to generate new drugs and treatments, Barry tells me. In the absence of a clear, granulomatous response upon which to model human disease, the second M has become a massive roadblock in the path to a cure. "The vast majority of the money that we spend in clinical trials based on mouse data is completely wasted," he says.
* * *
If you ask Clif Barry why we're still using the mouse to study tuberculosis, or Mark Mattson why we continue to test new drugs on obese and sedentary rodents, they'll tell you the same thing: Because that's what we've always done—we're in a rut. But to an outsider—say, a journalist who's trying to understand the place of the mouse in the broad enterprise of biomedicine—that explanation doesn't make sense. If you think of science as an industry of ideas, or a marketplace for medical technology, then there ought to be a clear and guiding incentive for greater efficiency in the lab—an invisible hand of self-interest with its fingers curled around every pipette and microtome. Any firm that finds a miracle drug can earn hundreds of millions in an IPO; any university professor who makes a breakthrough will gain fame and tenure; any disease that's cured may save countless lives. With so much on the line, how is it possible that all interested parties would be hamstrung by the same faulty method? Through what success or subterfuge did one particular rodent species earn its exalted spot among the three M’s of drug discovery? (Why should the second M stand for mouse, instead of monkey, marsupial, or mollusk?) In short: What's so wonderful about the lab mouse?
There's a standard answer to that question, one that can be repeated almost verbatim by biologists from across the spectrum of medical fields. The mouse is small, it's cheap, it's docile, and it's amenable to the most advanced tools of genetic engineering.
It's true that rats and mice are smaller, cheaper, and faster-breeding than many other mammals. Our last common ancestor lived 80 million years ago, which makes them closer cousins to us, in evolutionary terms, than either cats or dogs. (We share about 95 percent of our genome—although such comparisons tend to be more rhetorical than scientific.) And even among those who worry over the welfare of laboratory animals, there isn't much indignation at their use. (Rats and mice are denied many of the rights afforded other experimental mammals.) But a similar dossier could have been assembled in favor of any of a number of small creatures, some of which might be better-suited to answer certain questions. If you want to know about the visual system, for example, why use an animal that sniffs and whisks its way through the world? How about one that depends on its eyes, like a squirrel?
A rational calculation of its benefits and drawbacks misses the point of the modern lab animal. The real source of its influence, and the origin of its unique appeal to scientists around the world, is the simple fact of its having been chosen at all. Once we decided to focus our efforts on the rat and mouse, we learned how to fine-tune those species to fit our every need. When new technologies and methods entered the lab—improved products for animal care, better reagents for biochemistry, novel means for genetic manipulation—we tested them on, and tailored them to, our standard rodents. Meanwhile, the more rat and mouse data that accrued in scientific journals, the more tempting it was to devise follow-up experiments using the rat and mouse once more. In that sense, Barry and Mattson are right: We use these animals because that's what we've always done.
The feedback loop began more than 60 years ago, when federal investment in biomedicine was growing at an exponential rate. To eradicate the last vestiges of infectious disease, win the war on cancer, and otherwise mobilize the nation's resources for an industrial revolution in science, the government needed a more streamlined research model—a lab animal, or a set of lab animals, that could be standardized and mass-produced in centralized facilities, and distributed across the country for use in all kinds of experiments. An efficient use of federal research funds demanded an efficient organism for research.
In part because of their size and breeding capacity, and in part because they'd been used in laboratories since the turn of the century, the rat and mouse were selected for this role. As major research grants began to flow from Washington in the 1950s and 1960s, private rodent breeders picked up huge contracts with government-funded labs. Animal factories expanded rapidly throughout the Northeast, and then around the world. Lab mice were shipped out to the front lines of industrial medicine for cancer-drug screenings, radiation exposure tests, and even routine medical procedures. (Some early pregnancy tests required the injection of urine into female mice.) Rats, meanwhile, were the favored test-animals for industrial toxicology, and the norm in studies of behavioral psychology. Supply and demand surged upward in a spiral of easy breeding and cheap slaughter.
Standardized cages, food pellets, and husbandry techniques lowered costs. (A basic lab mouse now runs about $5 and can be maintained for a nickel a day.) Standardized suppliers made research more convenient. (A scientist can order animals for second-day delivery online, or by calling 1-800-LAB-RATS.) And standardized breeds—which ensure that every mouse or rat is a virtual clone of both its siblings and its ancestors—made it easier to replicate and verify studies from one lab to another.
This process reached its apotheosis 30 years ago, when decades of investment in the rodent model paid off with an extraordinary invention: The transgenic mouse. In December 1980, a group at Yale announced that it had injected a bit of foreign DNA into a fertilized mouse egg, and then implanted the embryo to produce a transformed but healthy offspring. Now the mammalian genome could be modified at will.
One discovery came after another: In '81, researchers at Cambridge managed to grow a population of embryonic stem cells for the very first time—again derived from the standard lab mouse. By the end of the decade, these had made possible an even more powerful research tool, the "gene knockout" mouse. Now we could design living animals with precise twists or snips in the curls of their DNA. Individual genes could be mutated, deleted, or added de novo, and a golden age of mouse research soon followed. Over the next 20 years—and running up through the present day—lab animals were engineered such that genes could be switched on and off in specific parts of their bodies, or in response to drugs placed in their drinking water, or light flashed through fiber-optic cables. Not only did these tools provide a better understanding of the genes and molecules associated with disease, but they led to new approaches to research and treatment—those involving human stem cells, for example—that might one day make lab animals of any kind obsolete. The possibilities were endless. What started as a lab animal had become the biologist's most magnificent tinker toy.
As the power of the mouse model grew, researchers found fewer and fewer reasons to study anything else. Even the stalwart lab rat began to seem antiquated and unnecessary. (Genetic tools for the rat have been much slower to develop; its first embryonic stem cells were created in 2008, almost three decades after the same was accomplished in the mouse.) In the 1990s, the National Institutes of Health placed the transgenic lab mouse squarely at the vanguard of biomedical research—the very model of the modern model animal. Under the leadership of Harold Varmus, who won his Nobel Prize for using chicken and mouse cells to identify the genes most susceptible to cancer-causing mutation, the NIH announced that Mus musculus would be the second mammalian species to have its genome sequenced, after the human. "This animal is one of the most significant lab models for human disease," announced Varmus.
The government continued to support work on other organisms, as it always had. "Everybody would prefer to work with the simplest, cheapest system that can yield good results," Varmus explains by phone. "There's no doubt that NIH is very sensitive to the need to help provide some kind of support for developing [new] models." In 1997, he led an NIH-wide effort to promote the study of zebra fish, a kind of minnow that grows as a free-swimming, transparent embryo. Now it's considered one of the most important models for research in developmental biology and several other areas. Varmus is also excited about new ways to study cells taken from human tissue, healthy or diseased, and cultured in a dish. But when it came to the genome project, and the most significant investment in living animal models for biomedical research, the mouse was a clear favorite. "It was chosen because not only was there a rich history of work," he says, "but because we knew how to do two important things: We knew how to add genes to the mouse germ line, and we knew how to manipulate genes that were in the germ line."
The extraordinary tools available to mouse researchers—including the ability to create transgenic animals and then stash their sperm and eggs in freezers so they could be reproduced at will—made it possible to envision a full catalog of gene knockouts, what Varmus calls an "encyclopedic project" in biomedicine. "Everything you do with a mouse is an approximation," he says, but scientists have now created tens of thousands of kinds of mice, each one designed to answer its own set of experimental questions. "We use these models in the hopes that we can recapitulate human disease, and create a playing field for trying to prevent and treat disease."
* * *
Microbiologist JoAnne Flynn has some concerns about mice, an inkling that in some fields, at least, we may be too reliant on the standard breeds. But if you ask her why she's been using them in her lab to study TB for the last 20 years, she has no trouble rattling off an answer: "They don't cough. They don't spread the disease. They live in small cages. They're easy to infect by any route. …"
I ask her to slow down so I can catch up in my notes.
"… You can take out the lungs of four mice, and get very similar data from each one. You can knock out any gene in the mouse, not in a guinea pig or rabbit. You can turn a gene on for a little while and then turn it off. You have every immunological reagent—all kinds of assays and antibodies. …"
In her biosafety-level-3 laboratory at the University of Pittsburgh, Flynn has used the standard animal model of TB to work out how particular elements of the immune system fight off the disease. In 1995, for instance, she applied knockout genes and monoclonal antibodies—two methods that were developed in the mouse—to show that a signaling protein called TNF-alpha helps mice survive an infection for months, instead of weeks.
"… You can have mice of different genetic backgrounds, and determine which genes make a difference. You can do vaccine studies. You can have cells that turn certain colors. You can make T-cells turn yellow when they do something. You can do trafficking studies, inject cells and watch where they go. You can collaborate with people to make new kinds of mice. …"
When she learned that doctors might prescribe TNF-inhibitors to patients with autoimmune diseases like rheumatoid arthritis, Flynn got in touch with one of the pharmaceutical companies making the drugs. If the mouse studies were right, she argued, then the treatment could be dangerous—especially for patients who were carrying latent TB.
In 2001, researchers at Boston University and at the Food and Drug Administration sifted through the database of adverse event reports for a popular TNF-inhibitor, and found more than five dozen cases of tuberculosis. The rate of infection among people taking the drug turned out to be four times higher than it was for other people of a similar health status. Twelve patients had died from the disease. Flynn was right. The results were published in the New England Journal of Medicine.
"… It may not be everything you want, but the mouse is a really flexible model," she says, finally taking a breath. Among her colleagues, the ratio of studies performed on mice to those using any other species of lab animal is about 50-to-1. "A mouse is an extremely reductive model, and it allows you to ask very specific questions," she continues. The role of TNF-alpha is just one of its many contributions to the study of tuberculosis: "The progress in TB research has been astronomical. But there has been a reckoning. People have had to stop and think, 'We're doing something wrong. We're not finding drug targets. We're not finding drugs that work the way we think they're going to work.' "
For Flynn, that reckoning came 10 years ago. She'd made a successful career working out the details of tuberculosis immunology in the mouse. But she knew that an effective, short-term treatment for latent TB—the form of the disease that affects roughly one-third of the world's population—wasn't going to emerge from studies like hers. The lab mice that she'd been using since her first job as a post-doc, and that nearly every other tuberculosis lab in the world had been using for decades, can only get an active infection. "That's a huge downfall of the mouse," she says. "I had to ask myself, am I missing something?"
If she wanted to study latent TB, she'd have to switch over to a new lab animal—the crab-eating macaque. Monkeys contract tuberculosis as humans do: Their lungs fill up with granulomas of different types and structures, and in 60 percent of cases they sustain the infection with no symptoms whatsoever. Converting her lab would be a daunting project, though. Monkeys are many thousands of times more expensive to keep around than mice, especially in the controlled environments used for the study of infectious disease. It takes longer to finish monkey experiments and publish monkey papers, which slows down the research as well as the process of getting grants and otherwise advancing your career. There's also the problem of small sample sizes, and the lack of genetic tools and reagents. Flynn went ahead with her plan nonetheless, and even after a decade's worth of monkey experiments she still spends some of her time rehashing facts about TB—such as the role of CD4 immune cells in containing the infection—that she'd established in the mouse years ago. These are data that many of her colleagues would have accepted without question. "It's incredibly risky and difficult for us," she says. "We took a leap."
Abandoning the mouse model may not be worth the time and money required, says Richard Chaisson, director of the Center for TB Research at Johns Hopkins University. "There are always questions about whether new drugs will behave in the mouse in the same way old drugs do, but so far nothing has come close to the accuracy of the mouse model for telling us what to expect in people," he explains in an email. "There is a great deal of interest in primate models right now, but there is no current evidence that this very expensive approach is as good as, let alone better than, the mouse."
Such evidence would be hard to come by. It's not clear how one might prove, in a satisfying and scientific way, that any given lab animal is better than another. We can't go back and spend the last 50 years studying monkeys instead of mice, and then count how many new drugs came as a result. The history of biomedicine runs in one direction only: There are no statistics to compare; it's an experiment that can't be repeated.
Before the mouse started to take over the field in the 1960s, the classical models for TB research were rabbits and guinea pigs—small, cheap mammals with a granulomatous response that's not too far off from what happens in humans. Since then, a number of new models have emerged: Goldfish and frogs develop a very similar disease, and so can the zebra fish, the minnow championed by the NIH: Its granulomas can be seen forming in real time. Flynn sees a place in the field for every one of these models. Different questions require different methods, she says. But if you want to study the course of latent TB in an animal host, than you're stuck with macaques or another nonhuman primate.
As for mice, "it's not that [they] aren't useful," she says, "or even that they're not the most useful possible system. Rather, it's that by focusing only on the mouse, we're running a grave risk."
For a scientist, switching lab animals in the middle of your career is something like changing religions. The academic world tends to cluster by model system: "Mouse people" talk to mouse people; "monkey people" talk to monkey people; each group exists within its own fibrous granuloma of meetings, conferences and review committees. When you submit a grant application to the NIH, or a manuscript to a scientific journal, the peers who assess your work are likely to be fellow travelers, members of your animal clique. Changing over from one species to another means backing out of a social sphere, and when the sphere you're leaving happens to be the biggest and best-funded, well: "I used to lose sleep over this," Flynn tells me at one point. And then later, with a chuckle: "I always say it's career suicide."
* * *
Clif Barry, the man of the Germantown menagerie, seems like he knows the value of standardization. When I meet him in his office at the National Institutes of Health in July, he's wearing a pair of black pants, a black belt, and black socks; a tight-fitting black polo shirt and a pair of black, rectangular eyeglasses from Armani Exchange; there's a pair of black sneakers beside him on the floor. The monochrome wardrobe makes life easier, he tells me. Everything matches everything else. It's one less thing to worry about.
Yet Barry has moved away from the standard model for tuberculosis research—the one that can be ordered by phone, hundreds at a time, in well-characterized strains and breeds. Like JoAnne Flynn, he's rejected the animal that allows for the most intricate molecular manipulations, and the most rapid screenings of new drugs. For him, the vaunted efficiencies of the murine-industrial complex are an illusion. Drugs go from mice to man, and then they fail.
In his own lab, he's spent the last three years working out what he calls an "advanced model" for drug-discovery—a brown-eyed primate with tufts of white poking from above its ears, and mottled, black-and-gray fur. At 7 inches in length, and weighing just half a pound, a marmoset isn't any bigger than a lab rat, and it reaches sexual maturity in half the time it takes a macaque. Around 90 percent of all marmoset births result in chimeric siblings with matching immune systems, which give Barry an easy way to control each experiment: It's like he's running a series of tiny twin studies. One marmoset gets a new drug and its littermate doesn't; then he compares the results. There's even hope that the marmoset might be susceptible to wholesale genetic engineering: In 2009, a Japanese team created a transgenic line of marmosets—the first time that had ever been accomplished in a primate species.
Compared with mouse research, though, Barry's advanced model moves at a glacial pace. His animals need 35 times more space than mice, and round-the-clock veterinary care. They're contagious, so lab workers have to wear puffy biohazard suits with built-in fans. And each animal must be trained laboriously to accept drug injections and crawl into a CT scanner. In all, says Barry, the switch to marmosets has reduced the number of compounds he can test in the lab by a factor of 50 or 100. "I'm continuously second-guessing it," he admits. Could a slower, less efficient animal model really help us cure tuberculosis?
Barry is betting that it can, but there's an eminence grise standing against him: 82-year-old doctor and microbiologist Jacques Grosset. A Frenchman with fluffy, white hair, Grosset has been training the next generation of TB researchers for the last half-century, screening new drugs and combination therapies in a strain of inbred mouse called the Bagg's Albino. They infect and inject hundreds of them at a time, in an ongoing parade of pale ears, pink eyes, and matching snow-white coats.
In the 1960s and ‘70s, Grosset participated in the development of the classic, four-drug treatment for TB; since then, he's run his mice through various tweaks and substitutions that might improve the therapy. Some of these have turned out more useful than others. In 1989, he observed that one of the ingredients in the cocktail seemed to be spoiling the mix: Infected lab mice were better off when he removed it altogether. Fifteen years later, Grosset's team made another big discovery: When they replaced the troublesome drug with a new one called moxifloxacin, their mice were cured in record time. "The findings suggest that this regimen has the potential to substantially shorten the duration of therapy needed to cure human tuberculosis," they wrote.
(Video: Lung scans from twin marmosets with tuberculosis. The sibling on the left suffers from a more virulent strain of the disease known as the "Beijing clade," which may be especially dangerous to humans. The distinction is invisible in mouse models.)
Barry considers Grosset a friend—he even named one of his frizzy-haired bichon frises Jacques in his honor—but he was skeptical when the moxifloxacin finding was announced at a lung-disease conference in Paris. Everyone was talking about how to get Grosset's new regimen into the clinic as quickly as possible, but there wasn't much reason to think the mouse data would translate to humans. "People were going wild; it was a frenzy. And I stood up at the microphone and said, 'This is a mistake. It's going to fail. And when it fails, I don't want to hear anything more about the mouse model.' "
No one listened. A major trial soon followed, enrolling more than 400 patients from 26 hospitals on four continents—the kind of research that typically runs tens of millions of dollars—and, sure enough, the results were disastrous. Swapping in the new drug had no observable effect on the course of treatment. When the scientists in charge, Grosset among them, published their report in 2009, they could only scratch their heads over "the apparent discordance between murine results and results of this clinical study."
A second group ran a very similar, and similarly expensive, clinical trial shortly thereafter, this time focusing on TB in American and Asian patients, rather than the Black Africans who predominated in Grosset's study. The results were more or less the same. "We keep getting led down the garden path," Barry argues. The doctors who devised the classic treatment 40 years ago didn't need detailed mouse data—they found their cure with a methodical, brute-force approach: a series of human trials that spanned the better part of two decades and tested every possible combination of exposures. "The way those four drugs were put together is incredible. It's never to be seen again."
Since that happened, we've had thousands of mouse studies of tuberculosis, yet not one of them has ever been used to pick a new drug regimen that succeeded in clinical trials. "This isn't just true for TB; it's true for virtually every disease," he tells me. "We're spending more and more money and we're not getting more and more drug candidates."
* * *
Clif Barry is not the only scientist frustrated by the pace of progress. It's not at all clear that the rise of the mouse—and the million research papers that resulted from it—has produced a revolution in public health.
It's hard to measure such things in aggregate, of course, but science and health policymakers have reached an uneasy consensus on this fact: We're at a moment of crisis in drug discovery. Last winter, current NIH director Francis Collins established a new institute (his agency's 28th) to address the "pipeline problem" in biomedicine: Despite pouring billions of dollars into research every year, our rate of innovation has slowed to a trickle. It takes more than a decade, and some $800 million, to produce a viable, new drug; among the compounds considered for testing, only 1 in 10,000 come to fruition.
These are grim statistics, the kind that get bandied about in discussions of health care reform and the national budget deficit. We've seen a revolution in molecular genetics, yet medical research has been spinning down to a halt. The United States spends more than twice as much on the health of its citizens as do most Western nations, yet ranks middling to poor on life expectancy, infant mortality, cancer survival rates, and many other important measures. No one knows why.
Some worry that with all our fine-tuned genetic methods, we've gotten hung up on the small-scale workings of disease. It's missing the forest for the trees, goes the argument: Doctors did better in the 1970s, when they tried to find drugs that worked in the clinic, regardless of the mechanism. Others acquit the basic science, but say our clinical trials—the human tests—are run so poorly that good drugs, safe and effective ones, are flunking out. Another group wonders if we're getting held up at the preclinical stage: Researchers may be using shoddy statistical methods in their animal work, or scrapping negative results, or even adding subtle bias to their data when they pluck certain mice from the cage instead of others.
Here's another way to explain the heavy expense and slow rate of return in biomedicine: Maybe the animals themselves are causing the problem. Assembly-line rats and mice have become the standard vehicles of basic research and preclinical testing across the spectrum of disease. It's a one-size-fits-all approach to science. What if that one size were way too big?
For some maladies, like tuberculosis, the rodent model may be inadequate to its core, lacking in the basic mechanisms of human disease. For others, the mere fact of the rodents' ubiquity—and the standard ways in which they're used—could be hindering research. Jeffrey Mogil, who studies chronic pain at McGill University in Montreal, points out that almost every datum of mouse research comes from male specimens, despite the fact that male and female mice respond to pain in different ways. Mark Mattson laments the factory breeding conditions and mass-husbandry practices that bias his experiments and leave his control animals overfed and unwell.
Perhaps the researchers have come to resemble their favored species: So complacent and sedentary in their methods, so well-fed on government grants, that any flaws in the model have gone unnoticed, sliding by like wonky widgets on a conveyor belt. It could be that the investigators and the investigated are locked together in the mindless machinery of science, joined hand and paw in the manufacture of knowledge. If there were something wrong with the rodent—not just its body weight or exercise habits, but in its fundamental utility as an instrument of learning—the scientists may not realize it at all.
It might take an outsider to see the problem, then, someone standing beyond the factory gates. Starting in the early 1990s, and coincident with the rise of the transgenic mouse, a set of historians and philosophers of science began to construct a formal critique of industrial biomedicine. They acknowledge, first of all, that the mouse and rat have been enormously productive, and that standardization brought with it unparalleled economies of scale. But they wonder whether a laboratory monoculture, with such a glut of cheap data, can be truly sustainable. Rats and mice were never so good at curing disease as they were at making data for its own sake: We have a million papers' worth already, and could soon have a million more: experimental results that might one day be mothballed in dusty stacks, next to those of some other research juggernauts—phrenology, miasmatism, radical behaviorism—that rolled along for decades before coming to a creaking halt, not so useful and not much cited.
Whether the critics are paranoids or prophets, the hordes of mice are marching on. Transgenic models have colonized the whole of biomedicine, and their influence grows daily. At a meeting in London last year, scientists announced the inevitable next step—an audacious plan to mutate every single gene in the murine genome and record the function of each one in a public database. It’s a massive, multigovernment undertaking that would reinforce the mouse's position as the most thoroughly examined and explicated higher organism on the planet—“a historic opportunity,” as one of its organizers, Mark Moore, describes it, “to systematically learn everything about a mammal for the first time.”
The project, which has the support of science agencies in the United States and Europe, will cost at least $900 million. That is to say it’s a monumental piece of megascience with a price tag on the order of the Large Hadron Collider. In the end, we’ll have an archive of stem cell lines—a community warehouse from which researchers can create (and order off the Web) an animal with any one of its 20,000 genes inactivated. And each of those myriad versions of the mouse will have been run through a standard battery of tests and had its essential features stored in a public database.
A bio-utopian dream like this can imprison us with grand expectations. Once you've invested so much into a single model, how do you know when that model's utility has run its course and it's time to move on? Vinny Lynch, an evolutionary biologist at Yale University, has a quixotic vision for the future of biology. It's the "hourglass model" of animal research: We started out in what was almost the random study of nature—a broad biology of birds and snails and elephants, uninformed by deeper understanding. Then came the narrowing of the 20th century, when all our research efforts were funneled through a slender sieve of model systems. Now we've reached the limit of that bottleneck—the inherent limitations of the mouse, let's say—and it's time to broaden out once more, to apply all the knowledge we've gained over the last hundred years to selecting new animals and new systems, and broaden out into a more rational, relevant science.
In a paper titled "Use with caution: Developmental systems divergence and potential pitfalls of animal models," Lynch makes the argument for entering this next phase. He tells me we may already have reached a tipping point, in fact. The mouse monopoly is teetering in the face of cheaper, faster genetic technologies. More and more species are having their genomes sequenced and their DNA manipulated in the lab. In a hamlet on the north shore of Long Island, the Cold Spring Harbor Laboratory keeps track of where we might be headed. Its Website declares, "the variety of organisms studied is currently undergoing a massive expansion." Among the emerging models profiled on the site are the wallaby and the wasp, the quail and the snail, the yam and the snapdragon.
These are encouraging indicators. They suggest that, in spite of the billion-dollar mouse mutation project now on the horizon, we may soon find ourselves backing away from the industry standards that have dominated biomedical research for so many decades. Clif Barry and JoAnne Flynn may be at the vanguard of a new movement within science, a retreat from the seductions of model organism-ism to something more diverse—a throwback, perhaps, to the slower, more comparative style of the 19th century, when theories were constructed from the differences among the many, rather than the similarities of a few.
Every standard organism has its limit, some finite store of knowledge that can be gleaned from it. There will come a point when we’ll have learned so much about the workings of a mouse, and dissected its every organ in such nanoscopic detail, that its veins of data run dry. There's a depth at which the science begins to founder, where its explorations become so profound and so specific, so lost in the letters of a foreign genome, that its reports to the academy no longer mean anything at all. To move beyond this dead end, to develop new treatments for disease and understandings of how it works, we'll need a more inclusive science, a more organic science, a research enterprise with room for mice and flies and worms, but marmosets, too—all the bugs and birds and beasts that live together in a vibrant ecosystem of discovery.
Part II: The Trouble With Black-6: A tiny alcoholic takes over the lab.
There's a warehouse about 20 minutes north of Boston, in a minor maze of buildings behind a barbed-wire fence. Ventilation ducts crisscross the ceiling over stacks of polyurethane pods, where half a million mice burrow in sterilized aspen, or stand up on their hind legs chewing irradiated food nuggets through wire-top lids. Up and down the aisles at the world's leading supplier of research animals, workers in solid-color scrubs—turquoise, purple, coral—stand on rolling stepladders with their arms inserted into the sleeves of one germ-free bubble or another, faces close up to the bulging plastic, manipulating the mice or moving them from cage to cage as if they were playing arcade games.
It takes five days for Charles River Laboratories to truck a crate of these animals from here to Seattle, and there are five dozen more facilities like this one around the world, with thousands of employees in 17 countries, shipping out millions of animals and earning some $700 million every year. The company sells research rodents in dozens of standard and specialized strains—mice, mostly, but also rats, and some rabbits, gerbils and hamsters, too. Their cheapest product, I'm told, is the CD-1 mouse, an all-purpose model for toxicology, aging, and oncology that goes for about $5. At the other end of the catalog are the custom-made mutants and the "preconditioned" models that have been surgically altered or otherwise seasoned for immediate use by the client. These cost as much as $400 each.
The best-selling animal, though—here and elsewhere—is a dark-coated, inbred mouse who's not too big and not too small, not too dumb and not too smart. He's called Black-6.
* * *
According to one estimate, distributors like Charles River and the scientists who buy from them have created at least 400 standard, inbred strains of mouse, and 200 inbred strains of rat. Yet one stands out from the rest as the model among models in biomedicine. If you want to set up a trading post for biology, a place where researchers from around the world can exchange and compare their data, then it helps to have a common coin—a stable currency that undergirds the system. In the global marketplace of discovery, the Black-6 mouse (more formally known as the "C57BL/6") serves as the U.S. dollar.
Almost 10 years ago, Nature magazine published the genome of the mouse—making it the second mammalian species after the human to have its DNA sequenced. But the researchers who conducted the work didn't use a field mouse caught from a barn, nor some unspecified laboratory strain. At a meeting held on Oct. 5, 2002, they debated their options, and decided by unanimous vote to sequence Black-6. It was on account of his "widespread use among the research community and favorable breeding characteristics," they wrote.
Yes, widespread: Just as mice and rats edged out the other lab animals in biomedicine, Black-6 has displaced the other mice and rats. Unless you had some reason to use one particular strain for your experiments—a nude mouse, let's say, for the screening of cancerous skin lesions, or the Bagg's Albino for its special role in the production of monoclonal antibodies—then Black-6 would be your fallback choice. The National Institute on Aging, for example, supplies 30,000 live, mature rodents every year to government-funded researchers around the country. A few years ago, Black-6 accounted for three-quarters of all the mice shipped out; now it makes up five-sixths.
Charles River won't break down its sales by strain, but a representative confirms that Black-6 mice outsell all others. A rival breeder estimates that Black-6 accounts for half to two-thirds of its orders. And the $900 million project to knock out and test the effects of every last gene in the mouse, to create a vast, public warehouse of these tens of thousands of mutant lines—that's being done in Black-6, too. When organizer Mark Moore says we have “a historic opportunity to systematically learn everything about a mammal for the first time,” he's not talking about just any mammal. He's talking about one that was created 90 years ago, when a mouse code-named C52 mounted one called C57 and deposited its semen in her reproductive tract with a waxy plug. Both were descended from a line of "fancy mice," sold as pets from a converted poultry farm in Granby, Mass., about 100 miles west of what now serves as the global headquarters for Charles River. That's where the Black-6 family tree took root.
"The more research you do on something, the more valuable it becomes," says geneticist and statistician Michael Festing, one of the world's experts on inbred lab mice. A format war hides in the history of biomedicine—a race to establish one mouse strain as the baseline. It didn't have to be Black-6, Festing tells me, but once the norm had been established, the costs of changing direction became prohibitive. "Really, it's just chance," he says. Use begat ubiquity.
So one dark-brown lab mouse came to stand in for every other lab mouse, just as the inbred lab mouse came to stand in for every other rodent, and the rodent came to stand in for dogs and cats and rabbits and rhesus monkeys, the standard models that themselves stood in for all Animalia. But where is Black-6 taking us? How much can we learn from a single mouse?
* * *
Jeff Mogil studies how rodents feel pain. He transforms their genes, constricts their nerves, inflames their paws and dips their tails in ice-cold water. He's a psychologist, a pharmacologist and a neuroscientist—one of many hoping to find new drugs for the one-third of the population whose trigger-happy neurons leave them in chronic discomfort. And like most of his colleagues, he's an expert—a connoisseur, even—of rats and mice.
Almost every pain researcher now uses one of two rodent breeds, Mogil tells me: Black-6 mice or Sprague Dawley rats. With each passing decade, the field has grown more devoted to this pair of animals, on the theory that at the molecular level, at least, mammalian species are more or less interchangeable—mouse to rat and rodent to ape. In 2008, Mogil conducted a survey of the PubMed database and found that rodent pain studies were about as popular as those of dogs, cats, rabbits, and horses through the 1970s. Thirty years later, they accounted for 92 percent of the research. "We're making this implicit assumption that things are the same in rat and mouse," he says, "and the reason we're making that assumption is because we're making another assumption—that if something is true in rats and mice, it's true in people."
In spring of the same year, Mogil decided to test the conventional wisdom among his colleagues, and so many others in biomedicine. If you look at all the genes that seem to matter for animal pain, how do they compare from one rodent to another? How similar are the pathologies for inbred mice and Sprague Dawley rats, at the level of their genes and proteins? "Frankly, we just published a paper that scared the hell out of me," Mogil says. According to his write-up in the August issue of Pain, many of the genes that were flagged as being relevant in one species didn't matter so much in the other. "Long story short: Things were completely different in the mouse."
The problem isn't limited to comparisons across rodent types. For a paper published in 1999, Mogil tested 11 different inbred mouse strains—albinos, white-bellied agoutis, dilute browns, Black-6—and ran them through a dozen standard tests of pain sensitivity. He found robust variation on every single measure. "That study made my career," he says. (He's now a named professor at McGill, and the recipient of copious research awards.) "At the time, the expectation was that all those strains were the same. Now everyone would say, 'Well, of course they're all different."
Amid all the data in that paper, one point stands out: No inbred line is more unusual in its response to painful stimuli, nor more of an oddball in the lab, than the one most favored by biologists. The Black-6 mouse turned out to be the greatest outlier in Mogil's tests, the ugly duckling. Put a few standard breeds of mice on a hot plate, and the Black-6 winces first. He's sensitive to cold, too, and analgesics don’t work on him as well as they might. "The geneticists have known for a long time that this is not an average mouse strain," says Mogil. "People don't want to deal with it. A lot of my career has been devoted to telling pain researchers that the simplifications they've made are too simple. The dirty little secret of C57BL/6 is that in a number of respects it's quite exceptional."
Mogil is the kind of guy who likes to tell "dirty little secrets." The peculiarities of Black-6 don't stop at the edge of the hot plate, he says. It's also one of the only strains of mice that consumes alcohol voluntarily, and it's prone to morphine addiction. There are other quirks, too, like its propensity for atherosclerosis and age-related hearing loss, and a strain-related fondness for nipping the hair off its cage-mates.
A few more facts fill out the profile of what's so often used as the "average" lab mouse. A recent study compared the immune systems of wild-caught mice to those of Black-6s raised in germ-free cages: Not surprisingly, the wild animals were more robust. Housing conditions on the factory floor leave most Black-6 mice (and other inbred strains) sedentary and overweight compared to their cousins in the field, and while a Black-6 can live for two or three years in captivity, it's typically studied or discarded within a few months of birth, to avoid unnecessary upkeep. Researchers also have a tendency to exclude female mice from testing—in Mogil's survey, almost four-fifths of pain studies were male-only—on the theory that estrus cycles might confound the data, or that including animals of both genders would increase the cost of their experiments.
There are plenty of good reasons to use Black-6, of course. He's a good breeder, he's not so hard to manage in his cage, and—in this era of the transgenic mouse—his dark coat helps out, too. To make a line of mutant mice, scientists mix a few stem cells from a light-furred breed called "129" into a Black-6 embryo, and then implant the embryo into a Black-6 female. When she gives birth, you can tell right away if the foreign DNA has taken hold: A transgenic pup grows up as a patchwork of 129 and Black-6, with tufts of cream or chinchilla fur sprouting through a coat of brownish-black. Nearly every transgenic line is made this way, with an altered gene from the 129 strain that’s diluted and back-crossed into a standard Black-6 background, like a swirl of peanut butter folded into a scoop of chocolate ice cream.
Yet it took a study like Mogil’s to point out a flaw that might have seemed obvious from the start: If you’ve chosen one breed of mouse—one flavor of ice cream—to serve as the vehicle for every genetic twist and the baseline for every study, then you’re stuck with all its idiosyncrasies. A standard is only as good as its quirks. The flavor of mouse that’s served up most often in the lab can be summed up in a single sentence: He's a teenaged, alcoholic couch potato with a weakened immune system, and he might be a little hard of hearing. For some applications, he's a sorry specimen.
* * *
The story of how one kind of mouse came to dominate the study of human health—and then, perhaps, to constrain it—begins a century ago, at a Gothic-revival building with flying buttresses and an iron weathervane across the street from Boston's Arnold Arboretum. It was there that a precocious Harvard undergraduate began a series of experiments on the genetics of purebred animals—mice that passed down every one of their traits from one generation to the next. His project would lead to the development of a major industry in breeding for profit, and promote an approach to science that relies on the essential similarity of all living things.
The Bussey Institution for Research in Applied Biology was teeming with biodiversity—"a veritable menagerie of rats, rabbits and guinea pigs," as one former member recalled, with a screech owl, a sparrow hawk, a tortoiseshell male cat, even a skunk. But Little's adviser, the geneticist William E. Castle, was interested in what all these animals had in common. Gregor Mendel's experiments on pea plants had just been rediscovered, and scientists now had the idea—described for the first time as genetics in 1906—that a single pattern of inheritance applied not only across the vegetable kingdom but to animals as well. Castle and his colleagues set to working out the finer details of this pattern by establishing some standard breeding lines—guinea pigs, rats, and rabbits, along with self-fertilized stalks of corn—in which an organism's particular genes could be traced and identified.
Little arrived at the Bussey in 1908, a square-headed track star still in his sophomore year, and started his own work with inbred mice. He purchased a few animals from the farm in Granby, and spent the next few years mating them brother-to-sister, for generation after generation, selecting only the most vigorous pups from each litter for another bout of incest. Eventually, the animals had become so entangled in their family ties as to be virtual clones of one another—a near-perfect line of identical twins, with identical parents and children, too.
In the first decade of the 20th century, no one knew how such experiments might play out. Some scientists worried that the process of inbreeding could never produce a viable stock, but Little's mice prospered in their pens at the Bussey, and went on to yield important early insights into the genetics of cancer. Within a few years, the inventor of the inbred mouse had an established career in academic science, dividing his time between the laboratory and the executive office, and serving as president of two major universities by the age of 37.
In 1929, he decided to create his own campus devoted to the mouse, and convinced a group of wealthy automobile executives to fund a research center at the edge of Acadia National Park in Bar Harbor, Maine. Little named the new laboratory after the head of a car company, Roscoe Jackson, and began applying the Detroit model to the manufacture of research organisms. His "thoroughbred" animals would become the raw material for biological research—as pure and reliable a subject of study, he promised, as the reagents on the shelf of a chemist. According to Karen Rader's excellent history of this project, Making Mice: Standardizing Animals for American Biomedical Research, the Jackson Lab began selling inbred mice in 1932, for 10 cents each plus the cost of delivery (with a money-back guarantee if the mice died in transit).
The first commercial catalog for lab mice listed nearly a dozen separate stocks for cancer research, some of which would become as standard as a screw thread. Among Little's early strains were the dark-furred C57BL mice that he'd created in 1921 using the Granby stock, and colonies of these made their way into nearly every lab at Bar Harbor. They had divided into at least 10 distinct sublines, remembered one of Little's early employees—but the most popular was No. 6.
* * *
Since the 1960s, the grounds at the Jackson Laboratory have been divided across the middle, with research and development on one side, closer to the pine-covered mountains, and mouse production on the other, in the direction of the harbor. The buildings are done up in the schlocky postmodernist style of 21st-century biotech—grids of turquoise glass and brick facade, as if a corporate office park had mated sloppily with Harvard Yard. In one factory building, workers stack crates of mice onto a conveyor belt, and slide them into climate-controlled trucks that make their way to 19,000 different scientists every year.
The earthy smell of food and feces fills the loading bay and seeps like a fog through hallways that run alongside enormous "barrier rooms," where JAX mice live out most of their lives in pressurized flow racks. Whatever germs are lurking on my clothes and in my hair might infect the colony if I got too close, but a journalist can watch the masked technicians through a window, working giant tweezers under darkroom lights. There are 5,000 cages in this particular room, 20 mice per cage—100,000 animals with their food nuggets and their water bottles and lite rock playing over a set of loudspeakers. Dolly Parton's "9 to 5" runs in the background as I make my tour. They just use your mind, and they never give you credit. It's enough to drive you crazy if you let it.
Jackson employs about 1,200 people here, mostly from Bar Harbor, and while we're in mind of sorting one strain from another, the workers do seem of a type: Ruddy, goateed, and prone to non-rhotic vocalizations. It's a fact that Clarence Little himself prized this spot on the Northeast Seaboard for its purebred locals: The population of Maine is "composed largely of the best New England stock," he once boasted to a reporter, and "nearly as homogeneous as is possible to obtain at the present time."
Like some other pioneers in the study of genetics, Little was a proponent of eugenics—he helped organize the Race Betterment Conference in 1928 and served as president of the American Eugenics Society in the following year. By the 1930s, he'd set his purifying impulse to mice, breeding them to be as interchangeable and "identical as newly-minted coins." After 40 generations of brother-sister matings, a feat that might be accomplished in a decade, a given strain of mouse will stabilize, its genes matched up in homozygous pairs. That's when each set of parents, and every son and daughter, becomes a perfect clone of one another, invariant over space and time. Through the window of the barrier room I can see Little's eugenic utopia realized, 400 generations later, in stacks of plastic shoeboxes—Black-6 pups born in 2011, but carbon copies, more or less, of their great-great-a-hundred-times-more-great-grandparents. Here in Maine, these mouse twins root around like living fossils, their genomes frozen in the roaring '20s.
It takes some effort, though, to stop evolution. A gentle-faced biologist named Bob Taft, who works a few buildings away, guards against genetic drift among the colonies. Given enough matings, even the best-kept inbred strains will start to shift and split, their base pairs jostled in a natural tide. In the four decades that followed the Jackson Lab's opening, at least 40 different sublines of Black-6 emerged by chance. Now it's Taft's job, as director of reproductive services, to keep this steady mutational drip off the barrier-room floor. He's supposed to ensure that today's Black-6 is the same as yesterday's, and tomorrow's, and the day after tomorrow's, too.
We enter a first-floor room lined with wheeled, metal thermoses that look like industrial garbage bins. Together these fridges contain about 3 million cryopreserved embryos—a library of microscopic mice, each smaller than the width of a human hair, stored at minus-196 degrees Celsius in cocktail straws stopped up with cotton. If I wanted to make a withdrawal, an infant from any of 5,400 mouse lineages could be defrosted, implanted, birthed, and weaned in about 12 weeks.
"I don't remember a time when I didn't know what a liquid nitrogen tank looks like," says Taft. He grew up on a dairy farm in northeast Ohio, and might have spent his life working there if it hadn't been for a wrist injury, the kind that makes it impossible to milk cows. So it was on to plan B, a graduate degree in reproductive biology, and a career spent mastering the nuances of animal IVF. (Here's one: "It's fairly easy to collect semen from a bull, but not always safe.") While Taft's cousins try to kill off the rodents that invade their farms, he's up in Maine making new ones as fast as he can—750,000 embryos added to the archives every year.
Mice get pulled from the archives, too. For the active strains—Black-6 and the others—resuscitation orders come down after every five generations of breeding in the barrier rooms. Taft defrosts a couple of males and females from the metal bin, and sends them over to repopulate the Jackson stock with disco-era genes. It's a matter of applying the efficiencies of industrial cattle farming to laboratory animals, he says. "I've been accused of trying to turn mice into small cows. I like to think we've been fairly successful."
* * *
From its start, the Black-6 was meant to be as reliable as a rubber stamp, but Little had grander aims for his identical, inbred mice. He wanted one kind of animal to stand in for all the others in the lab: A mouse that served as a tiny cow, sure, but also a rabbit, a dog and a monkey. His inbred legions would take the place of humans, too, in the testing of new drugs and treatments for cancer, tuberculosis, and every other disease of man or mammal.
When I finally see one of these tiny humans pulled from a cage by its tail, I don't know if it's a Black-6 from Jackson Lab or Charles River or some other rodent breeder. (Each sells its own version of the standard mouse, differing only by some slight degree of genetic drift.) Wherever it's from, the animal before me is about to be dropped into a large basin filled with milky water, where it will swim around for a few seconds, whipping its tail in a rodent panic, until it finds a platform hidden beneath the surface. Researchers use this setup, called the "Morris Water Maze," to test their mice for signs of cognitive impairment—a rodent analogue, perhaps, of what happens to people with Alzheimer's, Parkinson's, or schizophrenia.
After flopping around the tub for a bit, the mouse arrives at the platform and huddles up like a mini-Koosh with its whiskers twitching. It sits there a while, dopey and nitwitted, a lousy-looking stand-in for a human with his mind on the blink. But then it’s not long before what seemed so mean and pestilent starts to be familiar: a body shivering after a swim, with two eyes, two ears, a nose, and a mouth. The Black-6 has a tongue, too, and a throat and a stomach and a cluster of visceral organs wrapped up with veins and arteries; there are nipples on its chest and nails on its toes. Why shouldn't it stand in for a person?
"The greatest and, perhaps, radical distinction between different men's dispositions for philosophy and the sciences is this," wrote Francis Bacon nearly 400 years ago, "that some are more vigorous and active in observing the differences of things, others in observing their resemblances." The best kind of science emerges from the balance of these dispositions—an approach that begins by analyzing the parts, and then moves on to a theory of the whole. In August 1857, Darwin put things more simply: "It is good to have hair-splitters and lumpers," he declared.
With the rise of the modern lab mouse, the lumpers have come to dominate; their favorite tool was designed from the start to obscure the differences of things and champion their resemblances. Clarence Little began his inbreeding experiments at a time when the old-fashioned modes of hair-splitting—the comparative, exhaustive study of plants and animals—were in rapid decline. Biologists had made an astonishing run of observations about the sameness of things, starting with the discovery, by Theodor Schwann and Matthias Schleiden in the late-1830s, that every plant and animal on Earth comprises a set of cells, Nature's Lego bricks. A growing understanding of how each of these parts functioned revealed that every mammal and microbe might be reducible to a few standard molecules (nucleic acids, proteins, carbohydrates, and so on) interacting in a handful of ways. In 1926, just as Little's inbred lines of C57 mice were beginning to stabilize, a Dutch biochemist named Albert Jan Kluyver captured the lumper's credo in a famous phrase: "From the elephant to butyric acid bacterium—it is all the same!"
But the spread of Black-6 and the other inbred strains ran afoul of another tenet of the scientific method, articulated by one of Little’s contemporaries just as Jackson Lab was being established. In 1929, a physiologist named August Krogh, who’d spent his career studying glass worms, moth pupae, mice, grasshoppers, frogs, and many other species, declared that for many problems, "there will be some animal of choice, or a few such animals on which it can be most conveniently studied." To illustrate his point, he cited a variety of tortoise in which the trachea was unusually divided: "We used to say as a laboratory joke that this animal has been created expressly for the purposes of respiration physiology."
It’s an idea that remains current in science. Ask 100 biologists how they choose their animal models and they'll all say the same thing: First, you need to ask the right question, then you need to find the right means for answering that question. Rodent or fly, mouse or rat, Black-6 or Bagg’s Albino—when it comes to tinkering with Nature, there's a tool for every job. That's the rhetoric of modern science—that each experiment should have its most perfect method, a lab animal special to its purpose. We can joke, like Krogh, that the giant axon of the squid was created for the study of neural transmission, or that the optic nerves of the horseshoe crab were designed to be frayed and split for the study of vision, and Krogh's Principle lives on in an array of model species in use today: Armadillos for leprosy, prairie voles for autism, finches for language acquisition, leopard geckos and red-eared slider turtles for sex determination, sea slugs for learning and memory, and many more "animals of choice" for solving one problem or another.
In truth, the armadillos, prairie voles, and the other exotic models live only at the margins of biomedicine. For most questions that arise, the mouse offers at least some kind of a solution. It’s a skeleton key that’s tried at every one of Nature’s doors. If the Black-6 should happen to fall short—if its body or organs turned up wanting for one study or another—then we can tweak its genes until it works. Scientists now put human DNA in mice to make their brains deteriorate as if they had Alzheimer's, or to make their blood more vulnerable to leukemia, or to "humanize" their livers so they metabolize drugs like people do. In July, a team of scientists in Ann Arbor published the first successful implantation of a human anal sphincter into a transgenic rodent, a lab-made mouse with a man's ass that might one day help us find a cure for fecal incontinence. Eighty years ago, scientists could laugh at the idea of an animal created expressly for the lab. Today they do it all the time.
Even as these models proliferate, and new mice are drawn up to fit each field, the raw material remains the same. It may seem like diversity to have all these animals in the lab, so ingeniously devised for one purpose or another. Yet most are made from a common lump of clay. We've tried to turn a young, male alcoholic mouse into whatever we need him to be. He may be the most powerful, flexible organism in the history of biomedicine, but no matter what we do to him, no matter how far we twist his genes, he will always be Black-6.
* * *
Jeff Mogil is a hair-splitter. "People who study behavior are willing to live with more variability," the pain expert says over the phone from his office in Montreal. "It leads to a different mindset. Instead of measuring protein levels, I measure behavior. Sometimes incredibly stupid behaviors, like tail flicks or licks or blinks—but behavior. People who work in biochemistry,” though? They’re “more likely to be lumping."
Mogil has spent much of his career decrying the lumpiness of his field. The adoption of just a few standard animals—Sprague Dawley rats and Black-6 mice—used in a few standard ways has slowed down pain research and left millions of patients without useful drugs. By way of example, he points out that 70 percent of all sufferers of chronic pain are women, and most are middle-aged—yet the lion's share of what we know about the disease comes from juvenile male rodents. It’s a mismatch that has already wasted millions of dollars in failed clinical trials: In October 2002, a company called Endo Pharmaceuticals shut down one of its most promising drugs, MorphiDex, after finding that its remarkable effects in animals failed to translate to people. To Mogil, the problem was clear enough—a classic lumper’s error, repeated over the course of a decade and through a hundred published papers. When he ran his own study of the drug in 2004, he added one tiny wrinkle to make his point: Instead of testing MorphiDex on male Black-6 mice, as most labs would do, he used a combination of males and females. It turned out that Black-6 mice—and maybe rats and humans, too—have gender-specific ways of handling pain: What's accomplished by one receptor in the male seems to be handled by another in the female.
Several other pain treatments have failed in spectacular ways upon moving from the cage to the clinic. Drugs designed to block substance-P receptors and sodium channels succeeded in rodent models, but had little, if any, effect in people. According to Mogil, there's really just one commercial analgesic for human patients whose efficacy was first identified and tested in animal models—a derivative of cone snail venom called ziconotide—and it's not a particularly good drug.
With the help of transgenic mice, researchers have identified some 300 molecules that may play a role in pain or analgesia—a tenfold increase in potential drug targets since 1990. Yet the process of drug discovery continues to stagnate. The pipeline remains stopped up. "It's the dirty little secret of all of neuroscience," he says.
Mogil doesn't blame Black-6 mice and Sprague Dawley rats for the slow progress, exactly. He's more concerned with the ways in which they're used—the strains and genders, the models and assays, the hot plates and pinched nerves—all the things that yield plenty of data in the lab but may have little to do with what happens in the real world. Over the last decade, he's made a hair-splitter's tour of academic conferences, telling his colleagues that Black-6 mice are different from 129s, that mice are different from rats, that males are different from females, and that rodents are different from humans.
"People pretty much ignored it completely. They said, 'Everything is probably OK,' and they're probably right. I can't prove that anyone's findings are confounded," he says. For his own work, he continues to fax in his orders for Black-6 mice, getting a new box of animals sent over every three weeks from Charles River or Jackson Lab. It's one thing to point out the weaknesses of the method, and another to abandon it altogether. "If you can't beat 'em, join 'em. You can spit at the wind, but if it doesn't work, what are you going to do?"
Part III: The Anti-Mouse: Could a hairless African rodent be our secret weapon in the war on cancer?
In the outskirts of San Antonio, Texas, where guys dressed like the Marlboro Man loaf at gas stations and kids hang out at two-step dance halls, a sarcastic 55-year-old biologist from Zimbabwe is getting frustrated. She's only trying to do what thousands of her research colleagues do every week of the year. She's trying to make an animal sick. She wants to give cancer to a rodent.
There's a problem with the colonies of naked mole rats that Rochelle Buffenstein carted down to her new lab in Texas four years ago. Unlike mice, which die of cancer by the crateful, not a single one of her animals has ever developed a naturally occurring tumor. Nor has any other naked mole rat seen anywhere in the world.
If you want to know how cancer grows and how it kills, then it helps to have a case in front of you. Most researchers don't wait for tumors to pop up by chance: They irradiate their animals, or transplant tumors from one to another, or inject carcinogens directly into their bellies. In 2004, Buffenstein and her students tried one of these shortcuts. They placed some mole rats in a gamma chamber and blasted their pale, pink bodies with ionizing rays. The animals were unimpressed. When I visited Buffenstein’s lab this past July, many were still alive, skittering through the plastic tubes of their basement habitat at the Barshop Institute for Longevity and Aging Studies.
Four years later, Buffenstein tried again. This time she infected cells from a naked mole rat with a virus designed to corrupt their nuclei with the cancer-causing genes SV40 TAg and Ras. Then she slipped those cells into a live mouse, under the skin behind its ear. If you do the same using infected material from a mouse or a rat, or even a cow or a human, the transplant quickly grows into a deadly tumor, invading nearby fat and muscle tissue. But when Buffenstein and her colleagues used cells from a naked mole-rat, nothing happened.
That's how science goes: A hundred failures for every success. Earlier this year, one of Buffenstein's graduate students tried smearing the skin of half a dozen naked mole rats with a pair of vicious carcinogens: A synthetic compound called DMBA and an inflammatory agent known as TPA. When the same toxic pairing was applied to regular Black-6 lab mice as an experimental control, a cluster of tumors popped up within weeks. Every single mouse had cancer, and every single mouse died. The naked mole rats went on skittering through their tubes.
Her latest assault involves pouring carcinogens down the mole rats' throats in a last-ditch effort to induce liver or mammary cancer. But that may not work, either. For years, Buffenstein's laboratory Rasputins have been irradiated, poisoned, and heated up; their cells dosed with every imaginable pollutant—chemotherapies, oxidative stressors, and heavy metals—with little or no effect. "You name it," the professor says, "we tried all the kinds of toxins that are out there, and the naked mole rat seems to be very resilient and resistant." A drug regime that would be murderous to mouse cells must be doubled or tripled or even multiplied 50 times over, she explains, before it would have similar effects on a naked mole rat.
When Buffenstein takes me downstairs to see the animal colonies, we're not wearing gloves or gowns. At the massive rodent-breeding facilities in Massachusetts and Maine, where standard lab mice are manufactured by the millions, industrial technicians stalk around in what look like spacesuits, and the animals are housed in airtight isolator cages or ventilated barrier rooms that protect them from a list of 40 specific pathogens. Here in Texas, I've got a precious mole rat squirming, unprotected, in the palm of my hand. Its forebears were exported from a maze of burrows under a sweet-potato field in equatorial East Africa, but Buffenstein's not worried about my germs. I couldn't infect this animal if I tried.
The naked, 5-inch thing between my fingers, slightly bulbous at the hindquarters and topped with a set of lurid buck teeth, resembles nothing so much as a penis dentatus. Its pink skin, which feels as delicate as a moistened tissue, belies an astounding stamina. Mole rats have spent the last 24 million years adapting to a life spent in dark and humid tunnels six feet underground. Free of the terrestrial predators and frost that terrorize field mice, they've been left to wander down their own labyrinthine evolutionary path: In place of a mouse's fur, they have tiny hairs that brush against the walls to help them navigate; in place of a mouse's ears, they have a pair of pinprick holes on the sides of their heads; in place of a mouse's eyes, they have meager, malformed slits; and in place of a mouse's fragile constitution, the naked mole rat has what could be the most extraordinary set of natural defenses ever found in a mammal. A mouse's life is short and terrible—even in the lab, with plenty of food and a steady thermostat, it lasts for just three or four years at the most. A naked mole rat shows no sign of aging until it's a quarter of a century old. Blind and plump, it skitters around in a hazmat suit of its own creation.
That's why Buffenstein has gone to such lengths to nurture and sustain her exotic colonies, when she might just as well have filled her cages with bulk-rate mice from Charles River. Most biomedical researchers want to make animals sick so they can try to find a cure. Buffenstein does things the other way around. Instead of using rodents as a model of human disease, she takes them as a model of human health. Naked mole rats never get cancer; they cure themselves. She's determined to figure out how they do it.
In a paper co-authored for an animal research journal earlier this year, Buffenstein laid out the case for using the naked mole rat in the study of age-related chronic disease. With some work, she wrote, this animal could be something more than a quirky alternative to the mouse: a standard model on its own terms—a "supermodel," even, for certain fields of medicine. If you're interested in the progression of cancer or the biological mechanisms that promote its growth, she says, then a factory-made lab mouse might be your best option. "But as soon as you're looking at how to abrogate a process"—as soon as you start asking how we might go about preventing cancer in the first place, or slowing the decline of our bodies—"then I don't think [mice] are the right model at all."
It's conventional wisdom among scientists that the path to insight lies along a vertical plane, a descent into the details. "We are so ingrained to think that the best discoveries will come by studying in depth one species and not seeing the animal in a holistic perspective," says Buffenstein. Indeed, no mammal on Earth has been plumbed to such profundities as the inbred lab mouse. At some point, though, we may discover that we've been drilling in the wrong place—that other animals have secrets, too. Could the naked mole rat be hiding the most coveted one of all?
* * *
Among all the causes of human death, natural or man-made, cancer stands at No. 2. Nearly 570,000 Americans succumb to the disease each year—about one of every four deaths. That's despite a staggering outlay of research funding: More than $5 billion annually, and hundreds of billions spent in total since the passage of the National Cancer Act in 1971. Per capita, the United States government puts seven times more toward oncology research than the European Union, yet our practical gains have been modest at best, with mortality rates falling for some types of cancer (breast, colon) while rising for others (skin, liver). The War on Cancer looks like a quagmire.
The modern study of the disease, and search for a cure, has from its outset relied on armies of inbred lab mice, mobilized for the long engagement by policymakers in Washington. In 1938, the year after the founding of the National Cancer Institute, the feds designated Clarence Little's mouse factory in Bar Harbor, Maine as a central training ground for research animals. By the end of the 1940s, Little's Jackson Laboratory had more than doubled its mouse production, and soon hordes of cloned rodents were being marched to the front lines, injected or implanted with tumors, then bombarded with whatever chemical agents might shrink their tiny cancers. In 1974, a massive government research program was sifting through 3 million mice and more than 40,000 compounds every year. These same animals, purchased by the pallet from private breeders, dominate the field now more than ever: About three-quarters of all the recent animal cancer studies in the PubMed database draw their data from the mouse.
From the start, rats and mice had a distinct advantage over other model systems: They're small and cheap; they reproduce readily in the lab; their genes and lineage are easy to track. But the mouse, in particular, appealed to cancer researchers on account of its exquisite sensitivity to the disease. If a naked mole rat can't seem to get cancer on its own, a mouse can't seem to avoid it. Look too hard at a commercial Black-6, and it grows a lump: Seven out of 10 develop naturally occurring tumors. Other inbred mice are even more vulnerable, with spontaneous cancer striking more than 99 percent in some strains. (Wild mice, too, show a predisposition to cancer.) When Clarence Little hawked his factory-made organisms to the honchos at NCI in the 1930s, he was offering access to something more, or something less, than a mere laboratory animal. He'd created a line of cheap, disposable, tumor-making machines.
Consider that a man is 3,000 times bigger than a mouse; that he lives 40 times longer; that, as a result, his cells reproduce 100,000 times more often before he dies. To stave off malignancies, a human body must safely negotiate quintillions of chromosomal splits in a lifetime, versus mere trillions for a mouse. Yet a raft of genetic battlements prevent our nuclei from going haywire. Human cells, for example, have a built-in self-destruct mechanism that mouse cells lack—a molecular scissors that lops off bits of DNA with each go-round of the cell cycle. Cultured in a dish, human fibroblasts reach a natural endpoint—a state called "replicative senescence"—after around 50 cell divisions. For a would-be cancer to circumvent our natural defenses, and send a human cell multiplying out of control, it takes no fewer than six genetic mutations—six random, unlucky breaks. The same can happen to a line of mouse cells in just two steps.
What makes life harder for the mouse helps the scientist. By 1980, we knew of at least 1,600 chemicals that caused cancer in rodents, compared to just 15 for humans. Researchers could manufacture a cancer of the plasma cells by injecting a mouse's belly with mineral oil, or a colon cancer by feeding it aromatic hydrocarbons, or any of a panoply of other tumor types simply by infusing the right carcinogen into a mouse's bloodstream. An endless line of experimental subjects, cancer patients by proxy, could be made with chemical ingredients, or through other more direct means: Early drug-screening efforts implanted mice with leukemia or breast-cancer cells that had been sucked from the tumors of other mice; modern studies make use of the xenograft model, in which bits of human cancer tissue are placed into mice with poorly functioning immune systems. The most common vehicle for the latter—a flimsy, inbred strain that lacks a viable thymus—happens to be as pink and hairless as a naked mole rat. So much the better for watching tumors grow.
Nearly all of the cancer drugs we have today—some useful, others miraculous—came about as a result of this method. Modern chemotherapy regimens were devised according to a kludgy logic: Ply your rodent models with as many different chemicals as you can, then combine the best to find a drug cocktail that works (never mind why or how). A complementary approach, now in favor, takes more forethought: Researchers use transgenic mice and other tools to figure out exactly which of a cancer's genes and molecules make it thrive, and then try new drugs custom made to disrupt them. Whether you're using brute force or more targeted attacks, though, the path to the clinic runs through the mouse: Cages and cages of them, black ones and brown ones, albinos and nudes. It's a system designed for efficiency, designed to churn out tumors at maximum speed. But the industrial model of cancer research may only be slowing us down.
What's wrong with the lab mouse? The cancer it gets with such preternatural ease bears only a modest relation to our own. Mice are prone to lymphomas and sarcomas, the sorts of tumors that might crop up in blood or bones or cartilage. Humans tend to get carcinomas—cancers of the breast, the prostate, the lung, and the skin. While it’s true that certain rodent strains, like Black-6, have been bred to develop mammary lumps or other more humanlike diseases, these resemble rare, hereditary syndromes—an implacable family history of cancer, perhaps—rather than the sporadic cases that affect most people. Spontaneous cancers of the colon, bladder, stomach, or bronchi rank among the most common in humans but are virtually unknown in mice or rats.
Transgenic models may be limited in other ways. The molecular tweaks that can induce a tumor in the lab typically involve the wholesale deletion of certain genes, or their gross overexpression. Human cancers, by contrast, tend to result from more specific factors—one-for-one switches of nucleotides that affect some cells but not others, or alter a gene's function in subtle ways. Transplanting real, human tumors onto the bodies of mice gets around a few of these problems but introduces new ones. A xenograft rarely spreads within a mouse, so it can't really be used to study one of the most deadly aspects of human disease, metastasis. Nor does it provide an accurate picture of how a tumor might interact with healthy human tissue or coexist with the forest of bacteria that live inside our bodies.
All this adds up to a big problem: Mouse cancer as it’s studied in the lab has become as standardized as the animals themselves. In place of the organic and varied tumors that turn up in the hospital—a menagerie of cancers that evolve on separate paths within each patient—industrial science works with a bland and homogenized product, a fast-food version of the disease. A few years ago, Robert Weinberg, the MIT biologist who in 1982 discovered the first clear link between tumor-causing mutations in rodents and humans, told Newsweek's Sharon Begley that lousy animal models have become "the rate-limiting step in cancer research." It wasn't the first time he'd come out against xenografts and other rodent tools. In another interview, with Clifton Leaf of Fortune, Weinberg said that drug companies were wasting hundreds of millions of dollars on animal research that has little predictive value.
It's an old joke that scientists have cured mice of cancer a hundred times over, while humans continue to suffer. According to Derek Lowe, an acerbic chemist who works for the pharmaceutical industry and blogs at In the Pipeline, lab-made rodent tumors are well-known to be "horrible, just horrible" stand-ins for human disease. As with tuberculosis, for every cancer cure that makes its way from mouse to man, many more stop short of the clinic at great expense. No one in R&D trusts the animal models, writes Lowe, "as anything more than rough, crude filters on the way to clinical trials."
Few scientists would deny these flaws in the standard cancer models—yet fewer still would argue for an alternative. The mouse is the worst kind of lab animal, they say, except for all the others that have been tried. No organism does a better job of generating cancers; no animal provides more research value per dollar spent; no tool serves up more data for publication. What gaps remain between the mouse and human forms of cancer might one day be bridged with genetic engineering—soon we'll be running tests in "humanized" animals, embedded with bits of our own DNA. That's the dream, at least: Keep the strategy but refine the tactics. Having come this far, retreat would be unthinkable. So science will do what it always does: Go deeper, deeper, deeper.
* * *
Down in the basement, the mole rats are quiet; nothing is stirring. They cower in plastic boxes lined with shredded paper and linked up with 2-inch tubes. One timorous beastie finally noses its way out of the pile, and wriggles down a passageway toward a neighboring box. Until the 1890s, no specimen of these shy animals had ever been seen in the West, and they weren't studied in captivity until the 1960s. Even today, the husbandry of naked mole rats is more an art than an industry.
Everyone who cares for Heterocephalus glaber has her special formula; Rochelle Buffenstein's happens to taste like cream of wheat. She pours a few tablespoons of yellow powder into a bowl and stirs in some water to make a paste. Lab mice eat hard food pellets made from processed grains, minerals, and ground-up pork products. Naked mole rats—at least the ones in Buffenstein's lab—get "Pronutro," a South African breakfast cereal of corn, soy, and chicory. "It's designed for sportsmen," she says, proffering a spoon. "Maybe that's why the animals live so long."
Another theory: It's the chunks of fresh produce she leaves in their bedding every afternoon. At Charles River and the Jackson Lab, the mice nibble their pellets at any time of day. The mole rats in Texas enjoy a wholesome lunch: corn and green beans, yams, some fresh garlic or a chunk of banana. As we discuss their menu, I think of the factory mice in shoebox cages and their all-you-can-eat buffets. Mark Mattson, a senior scientist at the National Institute on Aging, calls the standard lab rodents "couch potatoes" and argues that feedlot rearing makes them unfit for medical research. The basement at the Barshop looks like an animal health spa by comparison: Between meals of fresh fruits and vegetables, the naked mole rats are free to scamper through a plastic maze and tussle with their siblings.
The stillness from before has by now subsided. The colonies are more comfortable with the humans in the room, and they resume their busy work: Inscrutable forays from one box to another, scored with high-pitched chirps. A mouse facility tends to silence (but for the sound of light rock piped in through speakers). Naked mole rats, once they've settled down, can deliver 19 distinct vocalizations within the human range of hearing.
The longer we stand there, the louder the chirping becomes, until another sound starts to take its place—a faint clicking noise that builds to a clatter. The mole rats have taken up the activity for which they're most ostentatiously designed: They're biting at the corners of the habitat with their momentous teeth. If one's not careful, the animals can chew their way out, says Buffenstein. (I've read elsewhere they can bite through concrete.) By this point it's getting hard to continue the interview. We have to raise our voices above the clack-clack-clack.
We step into another animal room, where Buffenstein keeps the naked mole rats' big, hairy relatives from Namibia. One of these—a Damaraland mole rat—slithers into a tube when we arrive and begins stomping its hind-paws on the floor, brushing them backwards. It's an alarm signal for the hive, and mole-rat siblings soon pick up the call, turning their bodies around and brushing their own feet in turn. This lighting of the beacons proceeds throughout the tubes for several minutes, until a scruffy platoon converges to defend the chamber beside my elbow.
Both naked mole rats and their Damaraland cousins live as ants or termites do, in a rigid, insectile society. A lone female produces all the offspring, while lesser castes forage, defend the colony, and care for her young. The naked mole rat queen, bigger and longer than her peers, stomps around and bullies her workers to keep them in line. In the other room, I saw one barge into a plastic box and muscle a scrawny male into what looked like a rodent 69. She was readying him to breed, Buffenstein told me; a queen usually takes between one and three consorts. I watched them squirm together, head-to-tail. It was obscene.
No other mammals are known to assemble like this, under a monarch's rule. Naked mole rats are even more bizarre for their smooth, pink skin (mammals have fur) and their enfeebled eyes (other mole rats see just fine). Like reptiles, they have trouble controlling their temperature (also strange; rodents are warm-blooded). As a matter of taxonomy, the naked mole rat is closer to a guinea pig or porcupine than a mouse or a rat, but really it's neither one nor the other. Buffenstein knows that she's working with an oddball; she did a lot of the work that proves it. "[The naked mole rat] does have very unique mechanisms that are not seen in other animals," she says, referring both to its superficial quirks and to whatever private biochemistry helps it to shrug off cancer, deflect toxic chemicals, ignore painful stimuli, and otherwise live five times longer than one might expect.
It's rare to hear a biologist indulge in such special pleading. The logic of model organisms depends, first of all, upon their essential blandness: If one life form is going to stand in for all the others, like a canvas that can be painted with disease, then crumpled up and thrown away, it ought to be bleached of unnecessary details. The perfect lab animal—the lumper's tool—would be uncontaminated by evolutionary oddities and stripped of genetic clutter: A dog that's not too doggy, say, or a rodent that's bred to be just average. That's another reason why scientists love to work with mice: They're boring.
Buffenstein pulls a Damaraland mole rat out of its cage so we can give it a closer look. There were mole rats on the farm in Zimbabwe where she grew up—"pests, as my father would call them"—but she didn't give them much thought until, as an undergraduate, she started collecting them in Kenya for research. Her post-doctoral work on bats, lizards, and kangaroos took her to Australia; at one point she rescued a baby 'roo from a fur trapper and carried it around for a while in her handbag. Even now, as a tenured professor at the University of Texas, Buffenstein's projects still have a bit of that fuzzy-edged, put-a-kangaroo-in-your-pocket feel. She tells war stories about her fellow mole-rat obsessives—people who schlep around the country, trading colonies for zoos, labs, and private homes. After picking up a batch in Arizona, her flight home was canceled, so she released the mole rats into the bathtub of an airport motel. The next morning, the cages were tucked under her legs like carry-on luggage and covered over with the financial section of the New York Times.
Aging and cancer research are hardly at the fringe of biomedicine, but Buffenstein's is a science of the weird. Where most of her peers get their animals prepackaged from industrial facilities, she takes hers straight off the farm—plucked from the soil, even—like heritage turkeys or heirloom potatoes. "I think [the use of mice] is constraining us to answer questions for which the mice are best-suited," she says. Her own laboratory rodent, as gnarly as a farm-stand fruit, offers an alternative. It's the anti-mouse.
* * *
Ten years ago, Buffenstein was one of just a handful of biologists studying naked mole rats in captivity; now her field comprises some three dozen labs around the world. Her colleagues have looked at why naked mole rats are immune to the pain caused by spicy foods, or how they avoid getting itchy when doused with histamine, or what allows their brains to get by without much oxygen and a shriveled pineal gland. In Rochester, N.Y., a pair of Russian-born biologists, Andrei Seluanov and Vera Gorbunova, are devoted to finding out exactly how naked mole rats keep from getting cancer. A couple of years ago, they announced they'd found a "clue."
The discovery grew from previous work, for which the husband-and-wife team had gathered tissue samples from a caboodle of rodent species, minced them up, and watched them grow in culture. (Some of the animals came from laboratory suppliers; others from local hunters, who shipped the carcasses to Rochester in Styrofoam boxes.) Recall that a normal human cell will split some 50 times before spinning down, while a mouse cell tends to divide with abandon. Seluanov and Gorbunova found that among their bigger rodents—the beaver, the porcupine, and the capybara—fibroblast cells behave like those from humans, reaching a senescent state that serves as a natural bulwark against cancer. But no such effect could be found in the naked mole rat, they claimed, nor in its similarly long-lived cousins, the grey squirrel, the chinchilla, and the chipmunk. These animals must have evolved some "alternative mechanisms" for keeping tumors at bay.
The Russians' follow-up paper, published the following year, zeroed in on just two species—the naked mole rat and the standard Black-6 mouse. The scientists scraped fibroblasts from the lungs and underarm skin of each and plated them in the lab. When they checked back a week later, the dishes of mouse cells had filled out into a dense layer of growth while the naked mole rat cells remained loosely packed. It's normal for cells to stop dividing once they're close together—in a process called "contact inhibition"—but the naked mole rat samples appeared to stagnate even when they were just barely touching. To Seluanov and Gorbunova, this suggested the presence of a novel backstop against cancer, one that had never been seen before in any species. A naked mole rat cell, they argued, is hypersensitive to crowds: The minute one of its cytoplasmic arms brushes up against a stranger, it stops dividing. A cell could be induced to overcome its shyness with the help of some cancer-causing proteins, and so emboldened it would grow out of control, like a tumor. But even then, a second line of defense would be activated: The cell would apoptose, or self-destruct.
If these findings hold up, it's not clear how they might be marshaled to improve human health, since the naked mole rat's system of "early contact inhibition" still wouldn't apply to human cells. But according to John Sedivy, a molecular biologist at Brown University whose editorial accompanied the naked mole rat paper, figuring out how extreme cancer resistance works in another animal might help us to devise some useful tweaks to our own cellular defenses. Instead of humanizing a mouse to find a better cure for cancer, then, we might try to prevent cancer by mole-ifying a person.
It's early work—and controversial—but Seluanov and Gorbunova won a prize from the journal that published the study: The Proceedings of the National Academy of Sciences named theirs the most important biomedical research paper of 2009. Even with this small triumph, Seluanov says he struggles to find support for his work. The committees that review grants for the National Institutes of Health tend to be "narrow-minded"; they don't like experiments on exotic species such as the naked mole rat and the gray squirrel. "The mouse is an excellent model, ideal for drug development. They gave us these powerful anticancer treatments—chemotherapy and gene therapy. But in order to prevent cancer, you need to look at organisms that are really cancer-resistant," he says. You need to do unconventional research using unconventional tools. "But for them, that's a big red flag."
Buffenstein has faced similar obstacles: "Even to this day, working in naked mole rats, it's quite difficult to get funding. As people at the [National Institute on Aging] keep saying to me, 'It's not that people in program management don't think it's a really exciting project, but the reviewers don't like the fact that you're critiquing the studies that they've done in mice.' "
The lack of dependable support from NIH makes life twice as hard for researchers like Seluanov and Buffenstein, whose work is already slower and more expensive than that of their peers. A cage full of naked mole rats costs four times as much to maintain as a cage full of mice, with fewer reagents and laboratory equipment available for purchase off the shelf or through supply catalogs. Nor can naked mole rat DNA be modified to the extent that's possible—or commonplace—for those using inbred mice. "I'm very envious of the fact that mouse models have transgenics," says Buffenstein.
The day before I arrived in San Antonio, scientists in Liverpool, England, announced that the genome of the naked mole rat had almost been worked out, one of the many special-interest organisms whose DNA is now being mapped with high-speed methods. (A more thorough accounting of the naked mole rat genome was published in Nature in mid-October.) This should help researchers find specific genes that are involved in extending lifespans or short-circuiting the growth of tumors. But there's little hope of generating "knockout" mole rats anytime soon. To make a transgenic Heterocephalus glaber, or even a knockout porcupine, scientists would have to know enough about those animals' reproductive biology to harvest their eggs, transform their stem cells, and then merge the two in a dish (before incubating and implanting the embryos). Even if all this could be accomplished—and keep in mind that it's been done in only a handful of species—the production of such animals would be painfully sluggish. To generate a reliable, transgenic line, researchers must breed through at least 10 generations. An inbred lab mouse can start reproducing 8 weeks after it's born; a naked mole rat or porcupine, on the other hand, doesn't reach sexual maturity until it's at least a year old.
That's why the government shies away from nonstandard research to begin with. Public money goes toward the kind of work that is most likely to deliver tangible results; it's invested at maximum return. A brand-new professor with her first R01 grant might buy a few cages of cancer-prone mice and start pressing out data, but someone who wants to study something unusual—motor control in the octopus, say, or the mating habits of a unisexual whiptail lizard—needs plenty of time to work out the basics, to set up her animal in the lab and describe what she sees. Buffenstein's list of publications stretches across many pages, including some of the top journals in the field, but her work on naked mole rats—20 years' worth, at this point—remains in its infancy, focused, to some extent, on first principles. "There's enough work to do in five lifetimes," she says.
The very thing that makes naked mole rats so interesting to Buffenstein—an astonishing vitality that lasts for decades—only makes her research more difficult. "You're caught between a rock and a hard place, because they live so long that your grandchildren have to finish the studies you start." Still, slow science may have rich rewards, and the decisions we make today—on whether to invest in new model organisms or build out the ones we already have—are sure to have profound effects on the (human) generations to come. Last year's announcement of a plan to create and phenotype every possible single-gene knockout of the Black-6 mouse—to build a $900 million community resource focused on the standard animal—may offer exciting opportunities for all those within the ever-expanding "mouse community." But with every dollar spent on digging deeper, with each new grant awarded for this continuing geology, the institutional inertia gets stronger.
The naked mole rat isn't the only promising yet untapped source of knowledge in biomedicine, nor are cancer patients the only ones who might be better served by a diverse approach to science. A few weeks ago, for example, a team of researchers in Boulder, Colo., announced it had found a set of natural chemicals in the Burmese python that might one day lead to new treatments for cardiac disease. A python uses these fatty acids to make its heart swell by 40 percent while digesting a meal. Since human hearts can also become enlarged, at times with disastrous consequences, snake biology might have something to teach us about the leading cause of death in the U.S.
For all their promise, neither the Burmese python nor the naked mole rat can exist on its own, as a stand-in for every other animal in the lab. When Buffenstein tries to give her mole rats cancer, she uses Black-6 mice as an experimental control. When the team in Boulder finally isolated its snake oils, it tested them in Black-6 mice. Even the pythons themselves were treated to the standard strains: Every other week, they got a single Sprague Dawley rat for dinner. To suggest that we don't need mice or rats for research would be as narrow-minded and absurd as saying that mice and rats are all we need.
Yet we seem to be headed further down the mouse path, with fewer chances to explore the side roads of discovery. The python researchers were denied NIH funding for their study, on grounds that it lacked relevance to human health. As Congress debates spending cuts for NIH in the face of a lingering recession, the urge to shrug off exotic animals will only get harder to resist. Politicians can laugh about studies of grizzly bears or fruit flies, but no one ever mocks the drab little mouse. The classic inbred strains, mobilized for the war on cancer many decades ago, may offer the certainty of data and the most efficient means of its production. But one thing is clear: To do battle against the killers of men, they can't march on alone.