For the past 15 years or so, astronomers have been collectively scratching their heads over Fast Radio Bursts, or FRBs: incredibly intense but also incredibly brief flashes of radio energy coming from seemingly random spots in the sky. They’re so fast—just milliseconds long—that it’s been very difficult to find out anything about them. Poof: They’re there, like a flashbulb going off, then they’re gone.
There’ve been more question than answers. Are they close by, or very far? What causes them? How often do they occur?
But now, after all these years, astronomers finally got the break they’ve been seeking. A new paper just published in Nature describes an FRB detected just last year, and a bit of sleuthing revealed its most critical characteristic: its distance. Turns out, it’s far away. Very far away.
The burst is called FRB 150418, so named because it was detected on April 18, 2015. It was first spotted by the Australian Parkes radio telescope as it was sweeping the sky, performing a survey to look for astronomical sources of radio waves. When the burst was detected, a rapid alert was sent out to other radio telescopes with higher resolution (and therefore able to better nail down the burst’s position on the sky). Within hours the Australian Telescope Compact Array was on it, pinpointing the burst’s location.
A day later, astronomers used the giant Subaru 8.2 meter telescope to observe that location in visible light, and found an elliptical galaxy sitting right at the burst’s position. They took spectra, determined the redshift, and found that the galaxy is a staggering 6 billion light-years away. That’s literally halfway across the visible Universe!
And right away one mystery was solved: (At least some) FRBs are not local. Not even close.
Follow-up observations found an afterglow, too, the fading light as the phenomenon decayed away. It took six days before it became too faint to detect.
What does all this mean?
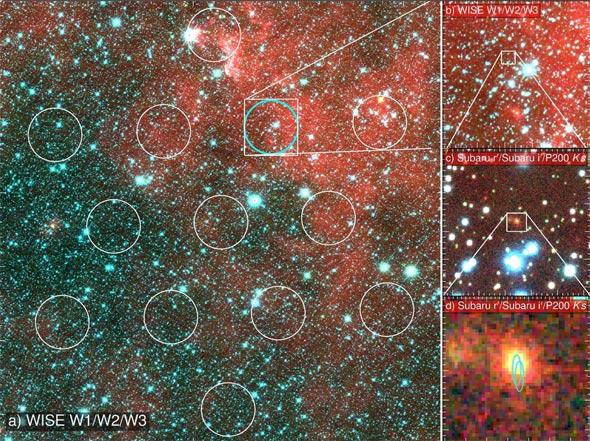
Keane et al.
The host galaxy of the FRB is an elliptical, which in general are old; no stars have formed there in a long, long time. That means whatever caused the FRB was probably not a massive star exploding as a supernova; those kinds of stars don’t live long after they’re born, and ellipticals don’t typically produce them. Also, supernovae tend to glow for weeks or months after the initial catastrophe, much longer than the weeklong FRB afterglow seen.
A more likely explanation is even more exotic: A coalescing pair of neutron stars. A neutron star is left over after a massive star explodes. The outer layers of the exploding star scream away, but the core collapses into a ultra-dense ball of quantum weirdness just a few kilometers across. If two such massive stars are a binary pair, orbiting each other, they eventually become a neutron star binary. Over billions of years, they spiral in to each other, merge, and form a black hole. The merger is incredibly violent and energetic, flinging out tremendous amounts of energy in a very short burst that may last only milliseconds.
That fits the FRB bill. And if any of this sounds familiar, that may be because there is a very close parallel here with gamma-ray bursts. They too are mysterious flashes of energy that were extremely difficult to nail down until technology got good enough to allow rapid follow-up. They were found to have afterglows, and studying those revealed them to be cosmological (very distant). There are two kinds of bursts: long duration, which can last for minutes, and short duration, which last for milliseconds … and which are thought to be due to merging neutron stars!
So it looks like at least some FRB and GRBs have something in common. More than that: They may be different flavors of the same kind of event.
But there’s more, and this part is really cool. As radio waves travel through the Universe, the ethereally thin amount of gas distributed through space changes them. The radio waves get dispersed, with higher energy (higher frequency) waves arriving a bit earlier than lower energy ones. It’s a bit like visible light passing through a prism and dispersing, creating the color spectrum, but the radio waves are dispersed in time, not space.
The amount of dispersion seen depends on how much stuff the radio waves pass through. But that doesn’t give you a distance; the source might be close by and passing through thick gas, or it might be much farther away and passing through much thinner material.
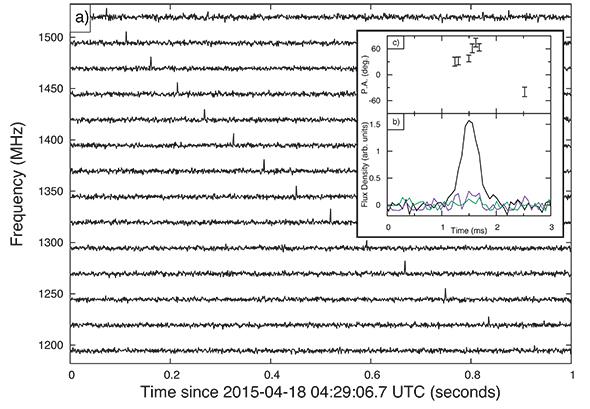
Keane et al.
But with FRB 150418, the distance was measured independently. With the total amount of material calculated through dispersion, and the distance known, the average density of material between us and it could be determined. This can then be compared to the current model of the Universe that predicts how much matter there should be … and they got a match!
Why is this a big deal? Because the Universe can be roughly divided into three components: dark energy (roughly 70 percent of the total mass/energy budget of the cosmos), dark matter (25 percent), and normal matter (5 percent). That last bit is us: regular old atoms, neutrons, protons, and the like. We’re very much in the minority here.
The thing is, we only see about half the normal matter in the Universe; the stuff “missing” is thought to be very hot gas distributed between galaxies but is very hard to detect. The observations of the FRB seem to show that the missing stuff isn’t so missing after all. The radio waves passed through it, were altered by it, and that change was measurable!
So, indirectly at least, the missing mass has been found.
And we’re not done yet. Once astronomers got a toehold on understanding gamma-ray bursts, we found out they come in a lot of different varieties. The story behind FRBs may be similar; lots of physical processes can create a short, powerful pulse of radio waves. We may yet find lots of different sources for this phenomenon.
And I wonder … with the recent LIGO detection of gravitational waves from merging black holes, in the not-too-distant future we may have more sensitive observatories, even some based in space that could detect gravitational waves from merging neutron stars. If that’s the case, then we’ll see a lot of fields in astronomy coming together. GRBs, FRBs, supernovae, galaxy collisions (supermassive black holes in their cores may collide as well), and more, all being observed in vastly different ways. Think of what we’ll learn!
One of the most fun aspects of science—and there are plenty to choose from—is that the mystery of yesterday is the observational opportunity of today. And the solutions we find always lead to more mysteries. Usually those are on a smaller scale once a phenomenon is first cracked, but as FRBs show us, there are still lots of undiscovered things going on in this Universe of ours. May they never end.